Study of postmortem brain demonstrates neuronal cell loss in the prefrontal cortex in autism
By Kris Dickson, Ph.D.
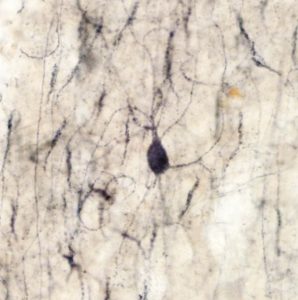
A chandelier cell in the prefrontal region of the brain in a donor with autism. The cell body is surrounded by cartridges (black) arranged in a parallel fashion. Image obtained from Martínez-Cerdeño/University of California, Davis
Much of the communication within the brain occurs through electrical signals that reflect both excitation and inhibition (E-I) of neurons. Changes in neuronal E-I balance have been implicated in a variety of neurodevelopmental conditions, including autism spectrum disorder (ASD)1,2.
A study published in the journal Cerebral Cortex earlier this year3 examined the postmortem brains of people with and without autism to better understand what leads to changes in E-I balance in ASD. In the study, Verónica Martínez-Cerdeño of the University of California, Davis and colleagues focused on a particular type of inhibitory neuron, called “chandelier cells.”
Chandelier cells have a characteristic shape resembling a chandelier, with long arms reaching out to contact excitatory neurons. Through these points of contact, each chandelier cell typically regulates the activity of hundreds of excitatory neurons4. So the loss of even a few chandelier cells could dramatically change the neuronal E-I balance that controls the communication of brain circuits.
Martínez-Cerdeño’s group had showed in previous studies5,6 that the brains of people with autism appeared to have fewer of a particular type of chandelier cell that produces the protein parvalbumin. But the method used in those studies did not allow the researchers to rule out whether in the brains of people with autism there were fewer chandelier cells, or whether the chandelier cells had less of the protein parvalbumin. While either change would ultimately impact brain circuit communication, shedding light on the ways in which chandelier cells are affected in ASD would be important to understand the mechanisms that drive brain and behavioral changes in ASD.
To resolve this issue, Martínez-Cerdeño’s team used another approach to quantify the number of chandelier cells in the prefrontal cortex of people with and without autism; instead of examining the body of the chandelier cells like they did in their previous studies, this time the researchers counted the ends of the chandelier cells. These parts of the chandelier cells are called “cartridges” and provide sites where these cells make contact and exchange signals with excitatory neurons.
In the new study, the researchers also relied on a different cohort of brain tissue, which included 11 brains from donors with autism and 11 brains from donors without the condition. These brains were obtained from the NIH NeuroBioBank, the CENE (Hispano-American Brain Bank for Neurodevelopmental Conditions) and the Autism Tissue Program, now Autism BrainNet.
Using the new method and brain samples, the researchers showed that the prefrontal cortex region of the brain of donors with autism had fewer cartridges than donors without the condition. This finding replicates the results from the previous studies, and provides further support for the possibility that an actual loss of chandelier cells may be affecting the activity of neural circuits in the prefrontal cortex in ASD.
The loss of chandelier cells is likely to cause a reduction in neuronal inhibition, which may lead to a brain circuitry that is overexcited, which in turn could result in sensory overload or even to epilepsy. Such findings may help explain changes in the brain activity of people with autism and provide a window into potential treatments. Further studies would be needed to understand what causes the loss of chandelier cells in the brain of people with autism, and whether other brain regions beyond the prefrontal cortex also have fewer of these cells.
References
- Sohal, V.S. and Rubenstein, J.L.R. Mol. Psychiatry, 24, 1248-1257 (2019) PubMed
- Rubenstein J.L.R., Merzenich M.M. Genes Brain Behav. 2, 255–267 (2003) PubMed
- Amina, S. et al. Cereb. Cortex 31, 2944-2951(2021) PubMed
- Markram, H. et al. Nat. Rev. Neurosci. 5, 793-807 (2004) PubMed
- Hashemi, E. et al. Cereb. Cortex, 27, 1931-1943 (2017) PubMed
- Ariza, J. et al. Cereb. Cortex, 28, 411-420 (2018) PubMed